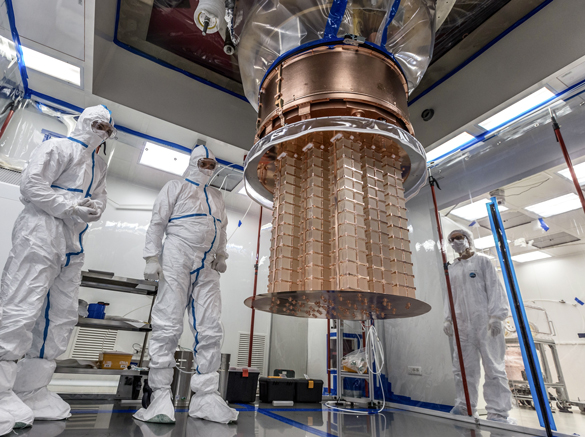
Researchers at the CUORE experiment (Cryogenic Underground Observatory for Rare Events) announced in Nature on April 6th new results on the nature of neutrino, which also demonstrate the exceptionality of the technology, capable of maintaining a detector weighing more than 700 kg at temperatures close to absolute zero, for more than three years.
CUORE operates in the cosmic silence in an experimental hall of the INFN Gran Sasso National Laboratories, protected by 1,400 meters of rock. It is among the most sensitive experiments in the world for the study of a nuclear process, called neutrinoless double beta decay, possible only if neutrino and antineutrino are the same particle. This decay, if observed, would clarify the mystery of the Majorana nature of the neutrino. The new results from CUORE are based on a data set ten times larger than any other high-resolution search with similar experimental techniques, collected over the last three years. Despite it phenomenal sensitivity, CUORE hasn’t yet seen evidence of neutrinoless double beta decay. Instead, CUORE has established that, on average, this decay happens in a single tellurium atom no more often than once every 22 trillion trillion years. The new limits of CUORE on the behavior of neutrinos are crucial in the search for a possible new discovery in particle physics, which would be revolutionary because it would help to understand our own origins.
“Ultimately, we are trying to understand the origin of matter,” said Carlo Bucci, researcher at the INFN Gran Sasso National Laboratories (LNGS) in Italy and the spokesperson of CUORE Collaboration.
“We’re looking for a process that violates a fundamental symmetry of nature,” added Roger Huang, a postdoctoral researcher at the Department of Energy’s Lawrence Berkeley National Laboratory (Berkeley Lab) and one of the lead authors of the new study.
"Neutrinoless double beta decay, if observed, will be the rarest process ever observed in nature, with a half-life more than a million billion times longer than the age of the universe,” said Danielle Speller, Assistant Professor at Johns Hopkins University and a member of the CUORE Physics Board.
CUORE is a scientific and technological triumph, not only for its new bounds on the rate of neutrinoless double beta decay, but also for its demonstration of the technology of its cryostat that operate in truly extreme conditions, at a temperature of ten-thousandths of a degree above absolute zero (10 milliKelvin). The temperature in the CUORE detector is carefully monitored with sensors capable of detecting a variation of just one ten-thousandth of a degree Celsius. The neutrinoless double beta decay has a specific energy signature and would raise the temperature of a single crystal by a well-defined and recognizable amount.
“It’s the largest refrigerator of its kind in the world,” said Paolo Gorla, a staff scientist at LNGS and CUORE's Technical Coordinator. “And it’s been kept at 10 mK continuously for about three years now.” Such technology has applications well beyond fundamental particle physics. Specifically, it may find use in quantum computing, where keeping large amounts of machinery cold enough and shielded from environmental radiation to manipulate on a quantum level is one of the major engineering challenges in the field.
"The extraordinary functioning of the CUORE cryostat is the culmination of a long technological challenge begun in the mid-1980s by Ettore Fiorini's group in Milan, which saw the evolution of cryogenic detectors, from crystals of a few grams to over 700 kg of the current ones”, added Oliviero Cremonesi, President of the National Scientific Commission for astroparticle physics at INFN.
“The resulting sensitivity of the detector is truly phenomenal: when there were large earthquakes in Chile and New Zealand, we actually saw glimpses of it in our detector,” said Laura Marini, a research fellow at Gran Sasso Science Institute and CUORE's Run Coordinator. “We can also see waves crashing on the seashore on the Adriatic Sea, 60 kilometers away. That signal gets bigger in the winter, when there are storms.”
CUORE is leading the way for the next generation of experiments: its successor, CUPID (CUORE Upgrade with Particle Identification), is already in an advanced stage of development and will be over ten times more sensitive than CUORE. “We’ll be operating until 2024, - said Bucci - I’m excited to see what we find.”
CUORE is operated by an international research collaboration, led by INFN in Italy and the Berkeley National Laboratory in the United States.
IN-DEPHT CONTENTS
Peculiar Particles
Neutrinos are everywhere — there are trillions of neutrinos passing through your thumbnail alone as you read this sentence. They are invisible to the two strongest forces in the universe, electromagnetism and the strong nuclear force, which allows them to pass right through you, the Earth, and nearly anything else without interacting. Despite their vast numbers, their enigmatic nature makes them very difficult to study, and has left physicists scratching their heads ever since they were first postulated over 90 years ago. It wasn’t even known whether neutrinos had any mass at all until the late 1990s — as it turns out, they do, albeit not very much.
One of the many remaining open questions about neutrinos is whether they are their own antiparticles. All particles have antiparticles, their own antimatter counterpart: electrons have antielectrons (positrons), quarks have antiquarks, and neutrons and protons (which make up the nuclei of atoms) have antineutrons and antiprotons. But unlike all of those particles, it’s theoretically possible for neutrinos to be their own antiparticles. Such particles that are their own antiparticles were first postulated by the Italian physicist Ettore Majorana in 1937 and are known as Majorana fermions.
If neutrinos are Majorana fermions, that could explain a deep question at the root of our own existence: why there’s so much more matter than antimatter in the universe.
A Rare Device for Rare Decays
But determining whether neutrinos are their own antiparticles is difficult, precisely because they don’t interact very often at all. Physicists’ best tool for looking for Majorana neutrinos is a hypothetical kind of radioactive decay called neutrinoless double beta decay. Beta decay is a fairly common form of decay in some atoms, turning a neutron in the atom’s nucleus into a proton, changing the chemical element of the atom and emitting an electron and an anti-neutrino in the process. Double beta decay is more rare: instead of one neutron turning into a proton, two of them do, emitting two electrons and two anti-neutrinos in the process. But if the neutrino is a Majorana fermion, then theoretically, that would allow a single “virtual” neutrino, acting as its own antiparticle, to take the place of both anti-neutrinos in double beta decay. Only the two electrons would make it out of the atomic nucleus. Neutrinoless double-beta decay has been theorized for decades, but it’s never been seen.
The CUORE experiment has gone to great lengths to catch tellurium atoms in the act of this decay. The experiment uses nearly a thousand highly pure crystals of tellurium oxide, collectively weighing over 700 kg. This much tellurium is necessary because on average, it takes billions of times longer than the current age of the universe for a single unstable atom of tellurium to undergo ordinary double beta decay. But there are trillions of trillions of atoms of tellurium in each one of the crystals CUORE uses, meaning that ordinary double beta decay happens fairly regularly in the detector, around a few times a day in each crystal. Neutrinoless double beta decay, if it happens at all, is even more rare, and thus the CUORE team must work hard to remove as many sources of background radiation as possible. To shield the detector from cosmic rays, the entire system is located underneath the mountain of Gran Sasso, the largest mountain on the Italian peninsula. Further shielding is provided by several tons of lead. But freshly mined lead is slightly radioactive due to contamination by uranium and other elements, with that radioactivity decreasing over time — so the lead used to surround the most sensitive part of CUORE is mostly lead recovered from a sunken ancient Roman ship, nearly 2000 years old.
CUORE is supported by the U.S. Department of Energy, Italy’s National Institute for Nuclear Physics (Istituto Nazionale di Fisica Nucleare, INFN), and the National Science Foundation (NSF). CUORE collaboration members include: INFN Gran Sasso National Laboratories, INFN Frascati National Laboratories, INFN Legnaro National Laboratories, INFN Divisions and University of Bologna, Genoa, Milano-Bicocca, Sapienza and GSSI Gran Sasso Science Institute in Italy; California Polytechnic State University, San Luis Obispo; Berkeley Lab; Johns Hopkins University; Lawrence Livermore National Laboratory; Massachusetts Institute of Technology; University of California, Berkeley; University of California, Los Angeles; University of South Carolina; Virginia Polytechnic Institute and State University; and Yale University in the US; Saclay Nuclear Research Center (CEA) and the Irène Joliot-Curie Laboratory (CNRS/IN2P3, Paris Saclay University) in France; and Fudan University and Shanghai Jiao Tong University in China.
NATURE ARTICLE:
https://www.nature.com/articles/s41586-022-04497-4
Contatti per la stampa:
Roberta Antolini, LNGS - Servizio Relazioni Esterne - Laboratori Nazionali del Gran Sasso: This email address is being protected from spambots. You need JavaScript enabled to view it.
INFN ufficio comunicazione
Antonella Varaschin, This email address is being protected from spambots. You need JavaScript enabled to view it.
REIS - 06.04.2022